Antimatter
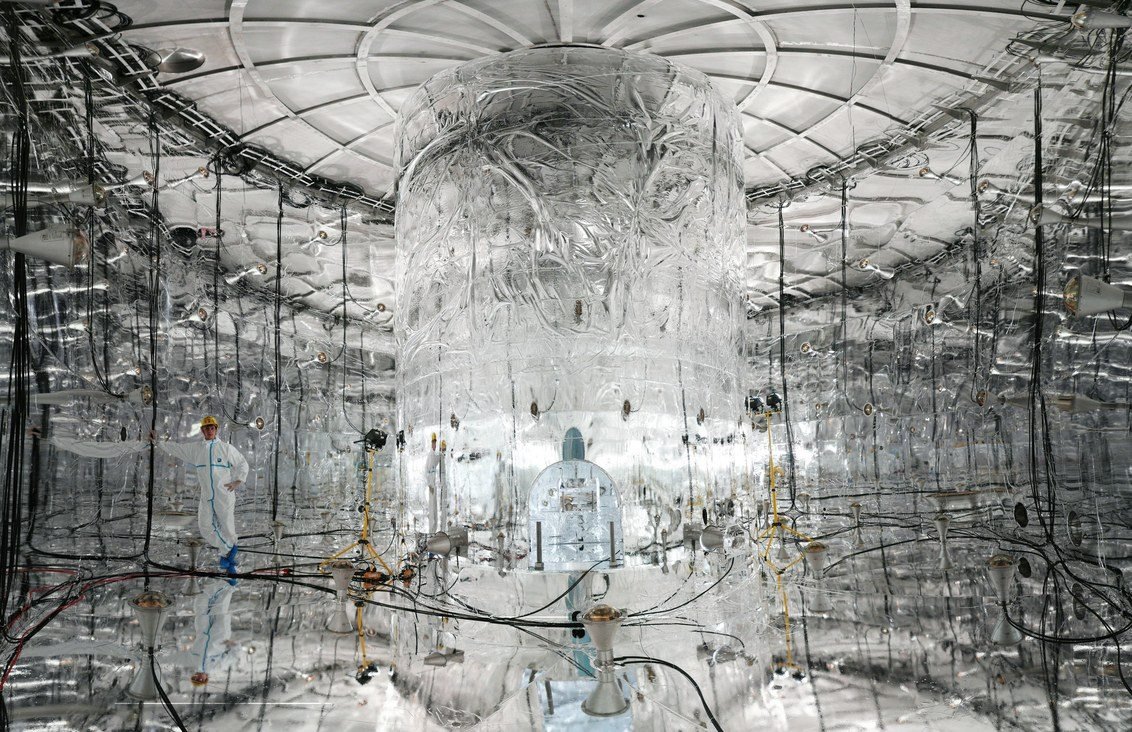
Researchers pasting a foil on the side of a hypersensitive detector designed to capture light
The first particles in the universe Formed after a hot and dense lump exploded. Physicists believe that in the extreme conditions of the Big Bang, light turned into matter: electrons, protons and neutrons, which later became part of us with you.
But physicists are not sure about exactly how such a transformation took place. In the 90s, physicists have shown that they can convert light into matter, pushing two radiation beams of extremely high energy. They also found that light at the same time creates an equal amount of antimatter. The very first particles of matter had to meet with their relatives from the field of antimatter and annihilate. Explosion – and there is no more matter.
But, obviously, matter is. For some reason, after the Big Bang of matter, more than antimatter has formed, and physicists do not know for what. “This is one of the biggest mysteries of the universe,” says physicist Don Lincoln of Fermilab.
Over the past 50 years, in laboratories and in equations, they hunted for processes that produce more matter than antimatter. One candidate is a predicted radioactive process in which two neutrons are converted into an atom into two protons. Theorists believe that in this process, known as the neutrinoless double beta decay, there are two electrons and no antimatter. Two new pieces of matter appear in the universe, and detectors should be able to detect them. If this process occurred many times after the Big Bang, he can explain where this additional matter came from.
But here’s the catch: no one has ever seen two neutrons transform into two protons. From previous experiments and calculations it is clear that this process is most likely to occur in certain atoms, for example, in germanium and xenon atoms. When two neutrons become protons in an atom of germanium, the atom turns into a new element, selenium. In a recent paper published in the journal Nature, researchers use data from their super sensitive detector to calculate that for half of the germanium crystal to degenerate into selenium, it will take more than 10 25 years. This is quadrillion times the age of the universe. “This is actually a very rare event,” says physicist Peter Grabmeier [Peter Grabmayr]one of the participants in the Germanium Detector Array experiment (GERDA) and one of the authors of the work.
]
Grabmeir is not afraid of such chances. To confirm that such a process takes place, it is not necessary to convert half of the crystal into selenium. It is necessary to detect the decay of only a few atoms. If any atom from their 36-kilogram germanium crystal turns into selenium, it will be able to detect the energy of the two emerging electrons, which will look like light in a collision with the detector. That other sources of radiation, such as cosmic rays, do not affect the detector, the germanium crystal was placed in a tank with liquid argon, at a depth of 1400 meters under a mountain in the center of Italy.
It remains possible that they will never detect this process , As Lincoln says. “But this is only an opinion,” he says. – I would not support it. I would not be surprised if such an experiment would refute my intuition. ”
In the meantime, physicists are exploring other processes that can explain the fact that the universe is made up of matter. In particular, they want to find all the differences of antimatter from matter, since any discrepancy can explain why their fates in the early universe turned out to be different. Last December, the Alpha experiment at CERN measured the properties of antihydrogen, but did not find any unexpected differences from hydrogen. In January, the Beauty experiment at the Large Hadron Collider discovered that when a particle called lambda-b baryon decays, its decay products do not fly at angles like its antimatter counterpart.
In the next ten years, Fermilab plans to build 1300 -km underground particle accelerator from Illinois to South Dakota – Deep Underground Neutrino Experiment (DUNE) [глубокий подземный эксперимент с нейтрино]. The goal of the experiment is to launch beams from neutrinos and antineutrinos over long distances, says Lincoln. If neutrinos behave differently than antineutrinos, it can help reveal another reason why there is more matter in the universe than antimatter.
These searches will be useful, even if nothing is found as a result, says Grabmeir . Their goal is to understand the rules by which the universe operates. If the process for which Grabmeier is ill does not exist, the fact itself can be used to exclude many of the hypotheses now proposed.
The Grabmeier Group plans to observe germanium and signs of radioactive decay for another two years. In the end, they want to use up to a ton of germanium in their detector. More germanium is more likely to see decay. “At some point we will find it,” says Grabmeir. But for now they are just waiting.
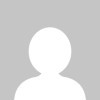